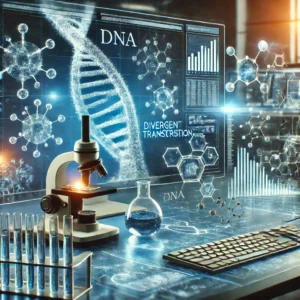
In the world of molecular biology, transcription is a fundamental process that shapes how cells function. Imagine it as the first step in turning a genetic blueprint into the proteins that perform vital tasks in the body. But for years, scientists believed this process was simple and one-directional. Now, groundbreaking research reveals a more complex story about how genes are transcribed and regulated.
For the past 25 years, the prevailing theory was that transcription happens in a unidirectional manner—meaning the genetic information is copied in one direction from the gene’s promoter. However, recent studies have upended this idea, revealing that transcription actually occurs in both directions from gene promoters, creating both stable messenger RNA (mRNA) and unstable, short RNA strands that don’t code for proteins.
But why does this happen? And more importantly, why does the body suppress these non-coding, antisense RNA strands? Let’s explore these fascinating questions and dive into the mechanics of how gene transcription works in eukaryotic cells—focusing on the latest insights from both yeast and mammals.
What Is Transcription and Why Is It Important?
Transcription is the process by which a gene’s DNA sequence is copied into messenger RNA (mRNA), which then serves as a template for building proteins. It’s a critical step in gene expression that determines cell function and identity. Misregulation of transcription can lead to diseases, from cancer to neurological disorders.
In the past, scientists believed that transcription proceeded only in the direction of the gene’s promoter. This straightforward process seemed simple enough: the gene would be copied into mRNA in one direction, leading to protein synthesis in the cytoplasm. However, this view has since evolved.
The Surprising Discovery of Divergent Transcription
Recent research has revealed a surprising twist in the transcription process. It turns out that RNA polymerase II (RNAPII)—the enzyme responsible for transcribing most genes—can initiate transcription in both directions from gene promoters. This means that both a stable, full-length mRNA is produced in the “coding” direction, while short, unstable RNA molecules are also created in the opposite, or “antisense,” direction.
These findings—first reported by Core et al. (2008), Preker et al. (2008), and Seila et al. (2008)—suggested that there’s a mechanism in place to regulate this process. Why would a cell produce this unstable antisense RNA (uaRNA)? What purpose does it serve? And how does the body ensure that the proper coding RNA is produced while preventing these aberrant antisense molecules from interfering?
Uncovering the Role of uaRNA: Characteristics and Mechanisms
The existence of uaRNA posed new questions. For one, what exactly are these RNA strands, and how are they different from the mRNA that codes for proteins? Through a series of groundbreaking experiments, we now know that uaRNA share many similarities with mRNA. Both are 5’ capped, suggesting they are processed similarly. They are also produced at rates similar to their sense counterparts, regulated by RNAPII pausing, and even elongated by the same factors involved in mRNA synthesis, such as P-TEFb.
However, there are some key differences. Unlike mRNA, which is stable and can be translated into protein, uaRNA are short—typically less than 1 kb in size—unstable, and quickly degraded by the RNA exosome, a cellular complex that ensures the removal of unwanted RNA. This indicates that uaRNA might serve as a transient regulatory element rather than a functional molecule.
How the U1-PAS Axis Regulates Transcriptional Directionality
So, how does the cell ensure that transcription occurs in the correct direction and prevents the production of unnecessary uaRNA? The key lies in the termination of transcription and the regulation of polyadenylation.
The research uncovered that the termination of uaRNA transcription is dependent on a sequence known as the polyadenylation signal (PAS). This signal causes the RNA to be cleaved and polyadenylated, marking it for degradation. The distribution of PAS and U1 binding sites in the DNA near gene promoters plays a critical role in determining the directionality of transcription. Specifically, an asymmetric arrangement of these sites regulates cleavage and polyadenylation near the promoter, helping to suppress the production of uaRNA in the antisense direction.
In essence, this mechanism helps maintain the integrity of the gene’s coding sequence, ensuring that only the correct, full-length mRNA is produced in the sense direction.
Conclusion: A New Understanding of Gene Transcription
The discovery of divergent transcription and the role of uaRNA has transformed our understanding of gene regulation. We now know that transcription isn’t as simple as it once seemed. It involves a complex dance of mechanisms that ensure both the stability and accuracy of mRNA production, while preventing unwanted transcription in the wrong direction.
These findings open up exciting new avenues for research, especially in understanding how the body controls gene expression at such a fine level. The mechanisms uncovered in this thesis suggest that the U1-PAS axis may play a broader role in regulating transcription outside of coding genes, offering new insights into how cells maintain their proper function.
As we continue to uncover the intricacies of transcriptional regulation, it’s clear that our previous understanding was only scratching the surface. The world of molecular biology is more complex—and fascinating—than we ever imagined.